People
Publications in scientific journals
Conference Reports
- Valavanides, M. S., Karadimitriou, N., & Steeb, H. (2022). Flow Dependent Relative Permeability Scaling for Steady-State Two-Phase Flow in Porous Media: Laboratory Validation on a Microfluidic Network. In SPWLA Annual Logging Symposium: Vol. Day 5 Wed, June 15, 2022. https://doi.org/10.30632/SPWLA-2022-0054
Other
- Krach, D., Ruf, M., & Steeb, H. (2024). POREMAPS 1.0.0: Code, Benchmarks, Applications. https://doi.org/10.18419/darus-3676
(Journal-) Articles
- Gao, H., Abdullah, H., Tatomir, A. B., Karadimitriou, N. K., Steeb, H., Zhou, D., Liu, Q., & Sauter, M. (2024). Pore-scale study of the effects of grain size on the capillary-associated interfacial area during primary drainage. Journal of Hydrology, 632, 130865. https://doi.org/10.1016/j.jhydrol.2024.130865
- Lee, D., Ruf, M., Karadimitriou, N., Steeb, H., Manousidaki, M., Varouchakis, E. A., Tzortzakis, S., & Yiotis, A. (2024). Development of stochastically reconstructed 3D porous media micromodels using additive manufacturing: numerical and experimental validation. Scientific Reports, 14(1), Article 1. https://doi.org/10.1038/s41598-024-60075-w
- Vahid Dastjerdi, S., Karadimitriou, N., Hassanizadeh, S. M., & Steeb, H. (2024). Formation of Common Preferential Two‐Phase Displacement Pathways in Porous Media. Water Resources Research, 60(12), Article 12. https://doi.org/10.1029/2024wr037266
- Wachsmann, S. B., Ruf, M., Prinz, C., Oehlsen, N., Zhou, X., Dyballa, M., Arweiler, C., Leistner, P., Steeb, H., Garrecht, H., Laschat, S., & Stegbauer, L. (2024). Chitin/Chitosan Biocomposite Foams with Chitins from Different Organisms for Sound Absorption. ACS Sustainable Chemistry & Engineering, 12(32), Article 32. https://doi.org/10.1021/acssuschemeng.4c00044
- Shokri, J., Ruf, M., Lee, D., Mohammadrezaei, S., Steeb, H., & Niasar, V. (2024). Exploring Carbonate Rock Dissolution Dynamics and the Influence of Rock Mineralogy in CO2 Injection. Environmental Science & Technology. https://doi.org/10.1021/acs.est.3c06758
- Taghizadeh, K., Ruf, M., Luding, S., & Steeb, H. (2023). X-ray 3D imaging–based microunderstanding of granular mixtures: Stiffness enhancement by adding small fractions of soft particles. Proceedings of the National Academy of Sciences, 120(26), Article 26. https://doi.org/10.1073/pnas.2219999120
- Ruf, M., Lee, D., & Steeb, H. (2023). A multifunctional mechanical testing stage for micro x-ray computed tomography. Review of Scientific Instruments, 94, 085115. https://doi.org/10.1063/5.0153042
- Dastjerdi, S. V., Karadimitriou, N., Hassanizadeh, S. M., & Steeb, H. (2023). Experimental evaluation of fluid connectivity in two-phase flow in porous media. Advances in Water Resources, 104378. https://doi.org/10.1016/j.advwatres.2023.104378
- Gao, H., Tatomir, A. B., Karadimitriou, N. K., Steeb, H., & Sauter, M. (2023). Reservoir characterization by push-pull tests employing kinetic interface sensitive tracers - a pore-scale study for understanding large-scale processes. Advances in Water Resources, 174, 104424. https://doi.org/10.1016/j.advwatres.2023.104424
- Lee, D., Weinhardt, F., Hommel, J., Piotrowski, J., Class, H., & Steeb, H. (2023). Machine learning assists in increasing the time resolution of X-ray computed tomography applied to mineral precipitation in porous media. Scientific Reports, 13(1), Article 1. https://doi.org/10.1038/s41598-023-37523-0
- Tatomir, A., Gao, H., Abdullah, H., Pötzl, C., Karadimitriou, N., Steeb, H., Licha, T., Class, H., Helmig, R., & Sauter, M. (2023). Estimation of Capillary-Associated NAPL-Water Interfacial Areas for Unconsolidated Porous Media by Kinetic Interface Sensitive (KIS) Tracer Method. Water Resources Research, 59(12), Article 12. https://doi.org/10.1029/2023WR035387
- Gao, H., Tatomir, A. B., Karadimitriou, N. K., Steeb, H., & Sauter, M. (2023). Effect of Pore Space Stagnant Zones on Interphase Mass Transfer in Porous Media, for Two-Phase Flow Conditions. Transport in Porous Media, 146(3), Article 3. https://doi.org/10.1007/s11242-022-01879-0
- Karadimitriou, N., and Marios S. Valavanides, Mouravas, K., Steeb, H., & and. (2023). Flow-Dependent Relative Permeability Scaling for Steady-State Two-Phase Flow in Porous Media: Laboratory Validation on a Microfluidic Network. Petrophysics – The SPWLA Journal of Formation Evaluation and Reservoir Description, 64(5), Article 5. https://doi.org/10.30632/pjv64n5-2023a4
- Kurzeja, P., & Steeb, H. (2022). Acoustic waves in saturated porous media with gas bubbles. Philosophical Transactions of the Royal Society. https://doi.org/10.1098/rsta.2021.0370
- Hommel, J., Gehring, L., Weinhardt, F., Ruf, M., & Steeb, H. (2022). Effects of Enzymatically Induced Carbonate Precipitation on Capillary Pressure–Saturation Relations. Minerals, 12(10), Article 10. https://doi.org/10.3390/min12101186
- Hasan, S., Niasar, V., Karadimitriou, N. K., Godinho, J. R. A., Vo, N. T., An, S., Rabbani, A., & Steeb, H. (2020). Direct characterization of solute transport in unsaturated porous media using fast X-ray synchrotron microtomography. Proceedings of the National Academy of Sciences. https://doi.org/10.1073/pnas.2011716117
Research
About this project
This research service project is an extension of the former Task Force Experiments. The projects starts in the second funding period of SFB 1313 and offers the chance that experimental methods and techniques are further centralised by creating a central experimental platform within the Porous Media Lab.
The project Z02 will (i) provide a cross-project experimental platform to produce results, either to be used in benchmarking, or as the means to provide input data to other projects. (ii) Develop new types of artificial porous media by using unconventional raw materials in combination with optical and soft lithography.
Additionally, independent research will be carried out related to the development of advanced experimental setups for applications complementary to those of the other projects.
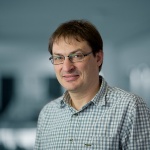
Holger Steeb
Prof. Dr.-Ing.Spokesman, Principal Investigator, Research Projects B05, C05, and Z02, Central Project Z
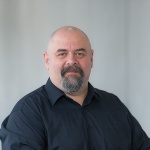
Nikolaos K. Karadimitriou
Dr.Principal Investigator, Project Z02 (PML)
[Image: Max Kovalenko]