People
Christoph Lohrmann (associated)
Dr. Simon Gravelle (Alumnus)
Kartik Jain (Alumnus)
Publications
Preprints
- Tovey, S., Krippendorf, S., Spannowsky, M., Nikolaou, K., & Holm, C. (2024). Collective variables of neural networks: empirical time evolution and scaling laws. In arXiv preprint arXiv:2410.07451. https://doi.org/10.48550/arXiv.2410.07451
- Tovey, S., Lohrmann, C., Merkt, T., Zimmer, D., Nikolaou, K., Koppenhöfer, S., Bushmakina, A., Scheunemann, J., & Holm, C. (2024). Swarmrl: building the future of smart active systems. In arXiv preprint arXiv:2404.16388. https://doi.org/10.48550/arXiv.2404.16388
- Gravelle, S., Beyer, D., Brito, M., Schlaich, A., & Holm, C. (2023). Assessing the validity of NMR relaxation rates obtained from coarse-grained simulations of PEG-water mixtures. In ChemRxiv preprint ChemRxiv:10.26434. American Chemical Society (ACS). https://doi.org/10.26434/chemrxiv-2022-f90tv-v4
(Journal-) Articles
- Schlaich, A., Barrat, J.-L., & Coasne, B. (2025). Theory and Modeling of Transport for Simple Fluids in Nanoporous Materials: From Microscopic to Coarse-Grained Descriptions. Chem. Rev., 125. https://doi.org/10.1021/acs.chemrev.4c00406
- Bursik, B., Stierle, R., Schlaich, A., Rehner, P., & Gross, J. (2024). Viscosities of inhomogeneous systems from generalized entropy scaling. Physics of Fluids, 36, Article 4. https://doi.org/10.1063/5.0189902
- Lohrmann, C., Holm, C., & Datta, S. S. (2024). Influence of bacterial swimming and hydrodynamics on attachment of phages. Soft Matter, 20, 4795–4805. https://doi.org/10.1039/D4SM00060A
- Schlaich, A., Vandamme, M., Plazanet, M., & Coasne, B. (2024). Bridging Microscopic Dynamics and Hydraulic Permeability in Mechanically-Deformed Nanoporous Materials. ACS Nano, 18, Article 38. https://doi.org/10.1021/acsnano.4c04190
- Tovey, S., Lohrmann, C., & Holm, C. (2024). Emergence of chemotactic strategies with multi-agent reinforcement learning. Machine Learning: Science and Technology, 5. https://doi.org/10.1088/2632-2153/ad5f73
- Gravelle, S., Beyer, D., Brito, M., Schlaich, A., & Holm, C. (2023). Assessing the Validity of NMR Relaxation Rates Obtained from Coarse-Grained Simulations of PEG-Water Mixtures. The Journal of Physical Chemistry B, 127, Article 25. https://doi.org/10.1021/acs.jpcb.3c01646
- Gravelle, S., Haber-Pohlmeier, S., Mattea, C., Stapf, S., Holm, C., & Schlaich, A. (2023). NMR Investigation of Water in Salt Crusts: Insights from Experiments and Molecular Simulations. Langmuir, 39, 7548–7556. https://doi.org/10.1021/acs.langmuir.3c00036
- Lohrmann, C., & Holm, C. (2023). Optimal motility strategies for self-propelled agents to explore porous media. Phys. Rev. E, 108. https://doi.org/10.1103/PhysRevE.108.054401
- Lohrmann, C., & Holm, C. (2023). A novel model for biofilm initiation in porous media flow. Soft Matter, 19, 6920–6928. https://doi.org/10.1039/D3SM00575E
- Tovey, S., Zills, F., Torres-Herrador, F., Lohrmann, C., Brückner, M., & Holm, C. (2023). MDSuite: comprehensive post-processing tool for particle simulations. Journal of Cheminformatics, 15. https://doi.org/10.1186/s13321-023-00687-y
- Gravelle, S., Holm, C., & Schlaich, A. (2022). Transport of thin water films: from thermally activated random walks to hydrodynamics. The Journal of Chemical Physics, 157. https://doi.org/10.1063/5.0099646
- Lee, M., Lohrmann, C., Szuttor, K., Auradou, H., & Holm, C. (2021). The influence of motility on bacterial accumulation in a microporous channel. Soft Matter, 17, 893–902. https://doi.org/10.1039/D0SM01595D
- Schlaich, A., Jin, D., Bocquet, L., & Coasne, B. (2021). Electronic screening using a virtual Thomas-Fermi fluid for predicting wetting and phase transitions of ionic liquids at metal surfaces. Nature Materials, 21, 237–245. https://doi.org/10.1038/s41563-021-01121-0
- Wagner, A., Eggenweiler, E., Weinhardt, F., Trivedi, Z., Krach, D., Lohrmann, C., Jain, K., Karadimitriou, N., Bringedal, C., Voland, P., Holm, C., Class, H., Steeb, H., & Rybak, I. (2021). Permeability Estimation of Regular Porous Structures: A Benchmark for Comparison of Methods. Transport in Porous Media, 138, 1–23. https://doi.org/10.1007/s11242-021-01586-2
- Zeman, J., Kondrat, S., & Holm, C. (2021). Ionic screening in bulk and under confinement. The Journal of Chemical Physics, 155, Article 20. https://doi.org/10.1063/5.0069340
- Breitsprecher, K., Janssen, M., Srimuk, P., Mehdi, B. L., Presser, V., Holm, C., & Kondrat, S. (2020). How to speed up ion transport in nanopores. Nature Communications, 11. https://doi.org/10.1038/s41467-020-19903-6
- Lee, M., Szuttor, K., & Holm, C. (2019). A computational model for bacterial run-and-tumble motion. The Journal of Chemical Physics, 150. https://doi.org/10.1063/1.5085836
Published data sets
- Bursik, B., Stierle, R., Schlaich, A., Rehner, P., & Gross, J. (2024). Additional Material: Viscosities of Inhomogeneous Systems from Generalized Entropy Scaling [DaRUS]. https://doi.org/10.18419/DARUS-3769
- Schlaich, A. (2024). Replication Data for: Bridging Microscopic Dynamics and Hydraulic Permeability in Mechanically-Deformed Nanoporous Materials [DaRUS]. https://doi.org/10.18419/DARUS-3966
- Gravelle, S. (2023). Molecular simulation scripts for slit nanopores with tunable hydrophilicity [DaRUS]. https://doi.org/10.18419/DARUS-3732
- Gravelle, S., Holm, C., & Schlaich, A. (2023). Molecular simulation scripts for bulk solutions [DaRUS]. https://doi.org/10.18419/DARUS-3179
- Gravelle, S., Holm, C., & Schlaich, A. (2023). Molecular simulation scripts for slit nanopores [DaRUS]. https://doi.org/10.18419/DARUS-3180
- Lohrmann, C., & Holm, C. (2023). Replication Data for: Lohrmann, Holm, Datta: Influence of bacterial motility and hydrodynamics on phage bacteria encounters [DaRUS]. https://doi.org/10.18419/DARUS-3836
- Lohrmann, C., & Holm, C. (2023). Replication Data for: Lohrmann, Holm: A novel model for biofilm initiation in porous media flow [DaRUS]. https://doi.org/10.18419/DARUS-3680
- Lohrmann, C., & Holm, C. (2023). Replication Data for: Lohrmann, Holm: Optimal motility strategies for self-propelled agents to explore porous media [DaRUS]. https://doi.org/10.18419/DARUS-3608
- Gravelle, S. (2022). Movies of thin film water on rough NaCl surface [DaRUS]. https://doi.org/10.18419/DARUS-2770
- Gravelle, S. (2022). Movies of thin film water on NaCl(100) surface [DaRUS]. https://doi.org/10.18419/DARUS-2697
- Gravelle, S. (2022). Molecular simulation scripts for thin film water on NaCl surface [DaRUS]. https://doi.org/10.18419/DARUS-2726
Research
About this Project
We investigate the flow structure and the porosity-permeability relationship of porous media that undergo morphology modifications due to evaporation or microbially induced calcite precipitation, as well as wettability modifications of the solid-fluid interface due to fluid pH, composition, or salt concentration changes. We develop simulation methods based on coupled multi-phase and multi-component Lattice-Boltzmann and molecular dynamics simulations, capable of reproducing these processes in detail on the pore scale, yielding results to be used to improve existing continuum models.
Results
Funding Period 1
In the first funding period, we established a multi-physics simulation framework by integrating a multiphase/multicomponent lattice Boltzmann method with electrokinetics (LB-EK). Using automatic code generation, we implemented the Shan-Chen pseudopotential model with tunable wetting and no-slip boundary conditions, and coupled it to a finite-volume electrokinetics solver to simulate dilute charged solutes interacting with multicomponent flows. In parallel, we developed an agent-based bacterial model capturing run-and-tumble motility calibrated to E. coli dynamics, as well as reversible surface attachment under flow (see Figure 1).
Funding Period 2
In the second funding period, we developed a precipitation model within the LB-EK framework, combining stochastic nucleation and crystal growth with an evaporation model to investigate salt crust formation. These simulations allowed us to analyze how wettability and capillary forces drive precipitation patterns, laying the groundwork for improved multi-scale integration. In parallel, we advanced biofilm modeling by coupling bacterial motility with flow fields and surface attachment mechanics (see Figure 2). These agent-based simulations revealed critical mechanisms behind early-stage biofilm formation and microbial transport efficiency in porous media, reproducing key experimental trends from research project C04. Atomistic simulations provided new insights into interfacial water dynamics and NMR relaxation behavior, supporting experimental NMRD data from research project C05 (see Figure 3). In addition, we collaborated with research project A01 on developing a generalized entropy scaling model by employing classical density functional theory, combined with a novel viscosity reference that accounts for the influence of solid–fluid interactions on fluid viscosity.
Fugure 3: Combining experimental and simulation approaches to study water dynamics in salt crusts. Fast-field cycling NMR and SEM imaging are complemented by molecular dynamics simulations measuring T₁ relaxation times near NaCl surfaces. Reprinted with permission from Gravelle et al., Langmuir, 2023, 39 (22), 7548–7556.
Future Work
In FP3, we will continue our effort to combine physical insights obtained on multiple scales in order to derive a multi-scale precipitation model for porous media. As a key contribution to the CRC’s salt vision, we analyse both the precipitation kinetics and the precipitate structure on a molecular scale. To further connect our simulations to experimental data we will continue our efforts to characterize experimental salt structures. This will be accomplished by bridging precipitation from the molecular to the pore scale by treating the solvent particles implicitly through machine-learned coarse-grained implicit solvent (MLCG-IS) potentials. Coupling precipitation to evaporation is tackled building upon our LB-EK approach, which we will refine using information from the molecular scale. Contributing to biomineralization benchmark, we will include pH variations for the induced precipitation for the urease induced urea splitting. We will further refine our biofilm model to study biofilm clogging in microfluidic experiments, in close collaboration with C04 and Z02.
For further information please contact
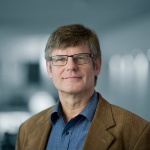
Christian Holm
Prof. Dr.Project Leader, Research Project C01